The results of the 2007 Phoenix Mars Lander Wet Chemistry Laboratory (WCL) analyses [1-4] clearly showed that a fundamental understanding of the habitability of any planetary body cannot be adequately obtained without direct knowledge of its aqueous geochemistry. By identifying a high concentration of perchlorate (ClO4-) in the soil, the WCL results helped explain the inability of several Mars missions to detect organics in the martian regolith, and generated a variety of hypotheses with implications for its geochemistry, habitability, and potential for supporting microbial life [5-6].
Likewise, determining the composition and properties of soluble species entrapped in the plume ejecta of Enceladus or the surface ice on an icy moon, is equivalent to the initial mineralogy studies that were performed on the surface materials of Mars. Evidence that these icy moons possess a subsurface liquid ocean in contact with a rocky core [7-8], directly impacts the hypothesis that they may be habitable and may support life [9]. Dissolved salts as measured by WCL would provide the strongest evidence for the types of water-rock interactions that are frequently invoked as necessary for habitability, and thus crucial for providing context for molecular biosignatures. Finding that subsurface oceans on Enceladus or Europa contain habitable environments would be of major scientific significance, regardless of whether or not life was detected.
The Enceladus Orbilander
Though the subsurface oceans of Enceladus are likely hundreds of kilometers below the surface, determining the nature of these habitats can be accomplished by analyzing the ejected plume material. The plumes of Enceladus are especially tempting because they are reasonably accessible and occur regularly [10]. As part of the the 2023–2032 Planetary Science and Astrobiology Decadal Survey (conducted by the National Academies of Sciences, Engineering, and Medicine for NASA) [11], a mission concept was proposed for a combination Enceladus lander and orbiter called the Orbilander (Figure 1) [12,13]. TheOrbilander would fly through the plumes and collect the ejected ice particles and would then also land on the surface to collect further in-situ samples of ice.
Figure 1. Proposed Enceladus Orbilander [12,13].
|
Figure 2. Plume ejecta accessable by Orbilander [12]. |
The Microfluidic Wet Chemistry Lab (mWCL)
Funded by NASA, we have developed a flow-through microfluidic WCL (mWCL) prototype shown in Figure 3. The mWCL is capable of determining similar species and properties as the Phoenix WCL, but of the ejected plume particles of Enceladus [13]. Using the heritage of the flight-proven WCL ion selective electrode (ISE) sensors, mWCL electrochemical sensor array will be able to characterize the aqueous chemistry of the plume ice particles by determining the presence and concentrations of soluble components such as; Ca2+, Mg2+, Na+, K+, Cl-, Br-, NH4+, SO42-, NO3-, CO2 (g) and O2 (g) and other dissolved gases, and chemical properties including pH, reduction-oxidation potential, alkalinity, and conductivity. The greatest challenge will be performing such analyses with the limited sample available from the plume.
Based on Cassini plume data [9,15], an estimate of the sample collected from the icy particle plumes of Enceladus by a reasonably sized collector with multiple flybys would be in the order of 50-100 μg. The mWCL must thus be able to analyze microliter-sized liquid samples. To allow for such µL-volume samples, the prototype mWCL was designed to hold 14 sensors with a channel size that would allow it to analyze a 100 μL sample. Thus the mWCL requires 104 times less sample than did the Phoenix WCL.
The prototype mWCL sensor array was calibrated using a variety of salt solutions expected on Enceladus with concentrations varying from 10-6 to 10-2 M. It was then characterized by performing analyses using simulants based on the known or modelled ocean chemistries of Earth and Enceladus, respectively. Selectivity coefficients were determined for the ISEs using the fixed interference method (FIM) and the matched potential method (MPM). With these values and the Nikolsky-Eisenman equation, we have shown that it would be possible for the mWCL to accurately measure the activities of soluble species in the presence of interferants and thus determine the ionic composition of Enceladus' oceans.
|
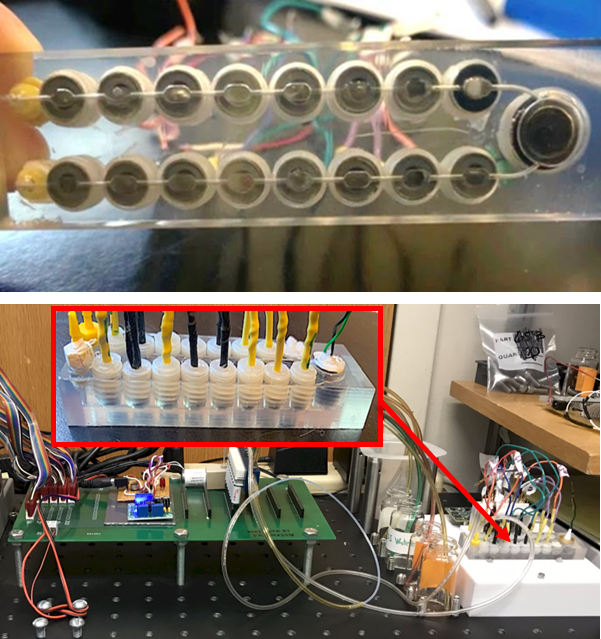
Figure 3. Prototype of the flow-through mWCL [13]. |
The Microfluidic Icy-world Chemical Analyzer (MICA)
In collaboration with NASA-Ames and JPL, and funded by a NASA grant, we are helping develop a more advanced version of the mWCL, the Microfluidic Icy-world Chemical Analyzer (MICA), for possible inclusion on the proposed Enceladus Orbilander [12,13] or other missions to Europa [16,17] or Mars. MICA (Figures 4 & 5) is a sample-processor-integrated, microfluidic implementation of the Phoenix WCL. It leverages and builds on: (1) four successful cubesat microfluidic bioanalytical payloads proven in Earth orbit; (2) a nanosat biopayload for deep space flight, to be delivered in 2021; (3) the microfluidic WCL (mWCL) and Sample Processor for Life on Icy worlds (SPLIce) NASA COLDTech projects
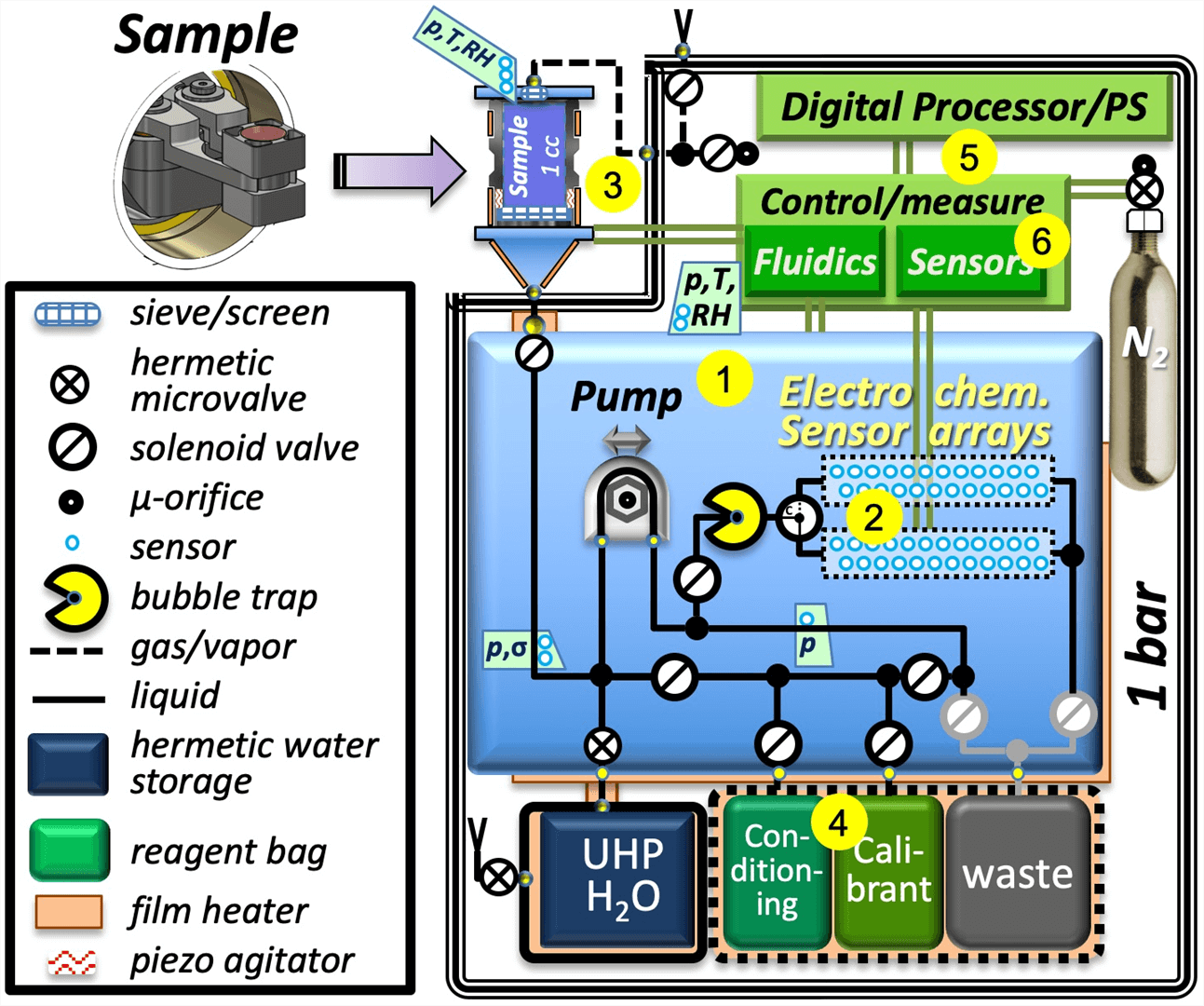
Figure 4. Functional block diagram of MICA showing (1) manifold, (2) dual sensor arrays, (3) externally-mounted sample-intake system, (4) reagent storage, (5) electronics, and (6) pressure and temperature measurement and closed-loop control [17].
|
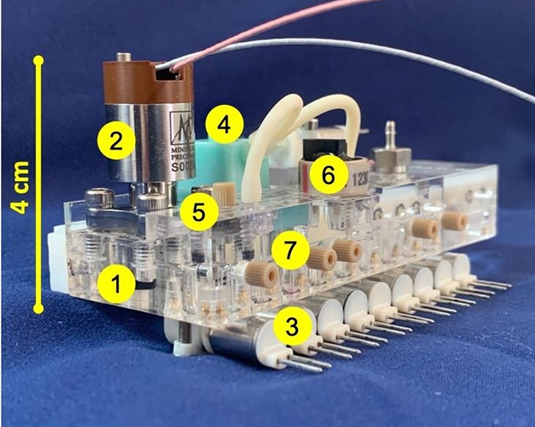
Figure 5. MICA fluidic manifold showing (1) fused 3-layer manifold; (2) microvalve; (3) 9 fluid-control valves; (4) peristaltic pump; (5) fluid-in-channel (AC conductivity) feedthrough; (6) piezoresistive pressure sensor; (7) liquid connectors [17].
|
MICA's electrochemical sensor array can determine the chemical energetics of surface or plume samples. It can measure dissolved gases and a variety of metal ions and can determine the electron-transfer properties that ccan reveal the energetic differences necessary for life. MICA is being developed primarily to provide soluble-chemistry context, habitability information, and comparative oceanography data as an element of a payload instrument suite on search-for-life missions, such as the proposed Europa Lander. The microfluidic system supports sample volumes from a few µL to many mL. It carries ultrahigh purity water and freeze-dried conditioning reagents and standards to prepare and calibrate sensors, which are configured as a pair of redundant, fluidically isolated sets of 24 sensors each with multiple reference electrodes. Replicate measurements will be made on multiple aliquots of each sample; samples are readily diluted by the microfluidic system so that high-concentration ionic species can be measured beyond the typical ~ 105 dynamic range (~ 5 µM - 1 M) of most of the ISEs [17].

[1] Kounaves, S. P., et al. (2010) Wet Chemistry Experiments on the 2007 Phoenix Mars Scout Lander: Data Analysis and Results, J. Geophy. Res., 115, E00E10. Full Text
[2] Kounaves, S. P., et al. (2014) Identification of the Perchlorate Parent Salts at the Phoenix Mars Landing Site and Possible Implications, Icarus, 232, 226-231. Full Text
[3] Kounaves, S. P., et al. (2010) Soluble sulfate in the martian soil at the Phoenix landing site, Geophy. Res. Lett., 37, L09201. Full Text
[4] Quinn, R. C., Chittenden, J. D., Kounaves, S. P., Hecht, M. H., et al. (2011) The oxidation-reduction potential of aqueous soil solutions at the Mars Phoenix landing site, Geophy. Res. Lett., 38, L14202. Full Text
[5] Stoker, C. R., Zent, A., Catling, D. C., Douglas, S., Marshall, J. R., Archer, D., Clark, B., Kounaves, S. P., et al. (2010) Habitability of the Phoenix landing site, J. Geophy. Res., 115, E00E20. Full Text
[6] Carrier B. L. & Kounaves, S. P. (2015) The origins of perchlorate in the Martian soil, Geophy. Res. Lett., 42, 3739-3745. Full Text
[7] Postberg, F., et al. (2011) A salt-water reservoir as the source of a compositionally stratified plume on Enceladus, Nature, 474, 620-622. Full Text
[8] Postberg, F. et al., ( 2009) Sodium salts in E-ring ice grains from an ocean below the surface of Enceladus, Nature, 459, 1098-1101. Full Text
[9] Choblet, G., et al. (2022), Enceladus as a potential oasis for life: Science goals and investigations for future explorations, Experimental Astronomy, 54(2), 809-847, doi: 10.1007/s10686-021-09808-7. Full Text
[10] Hansen, C. J., et al. (2006) Enceladus' Water Vapor Plume, Science, 311, 1422-1425. Full Text
[11] National Academies of Sciences, Engineering, and Medicine (2022), Origins, Worlds, and Life: A Decadal Strategy for Planetary Science and Astrobiology 2023-2032. Washington, DC, The National Academies Press. https://doi.org/10.17226/26522. Full Text
[12] MacKenzie, S. M., et al., (2021), The Enceladus Orbilander Mission Concept: Balancing Return and Resources in the Search for Life, Planet. Sci. J, 2(77), doi: 10.3847/PSJ/abe4da. Full Text
[13] MacKenzie, S. M., et al., (2022), Science Objectives for Flagship-Class Mission Concepts for the Search for Evidence of Life at Enceladus, Astrobiology 2022, 22, 685-712, doi:10.1089/ast.2020.2425 Full Text
[14] Kounaves, S. P., et al., NASA/ESA 16th Int. Planetary Probe Workshop, 8Jul2019, Oxford, UK. Full Text
[15] Yeoh, S. K., et al. (2015) On understanding the physics of the Enceladus south polar plume via numerical simulation, Icarus, 253, 205-202. Full Text
[16] Noell, A., Jaramilllo, E. A., Kounaves, S. P., et al., MICA: Microfluidic Icy-World Chemistry Analyzer, AbSciCon, 24-28 June 2019, Bellevue, WA Absract # 408-7. Full Text
[17] Ricco, A. J., Kounaves, S. P., et al., MICA: Microfluidic Icy-World Chemistry Analyzer, AGU Fall 2020 Meeting, 1-17 December 2020, Poster P044-0001. Full Text
|